Calculating Dg From Dh And Ds
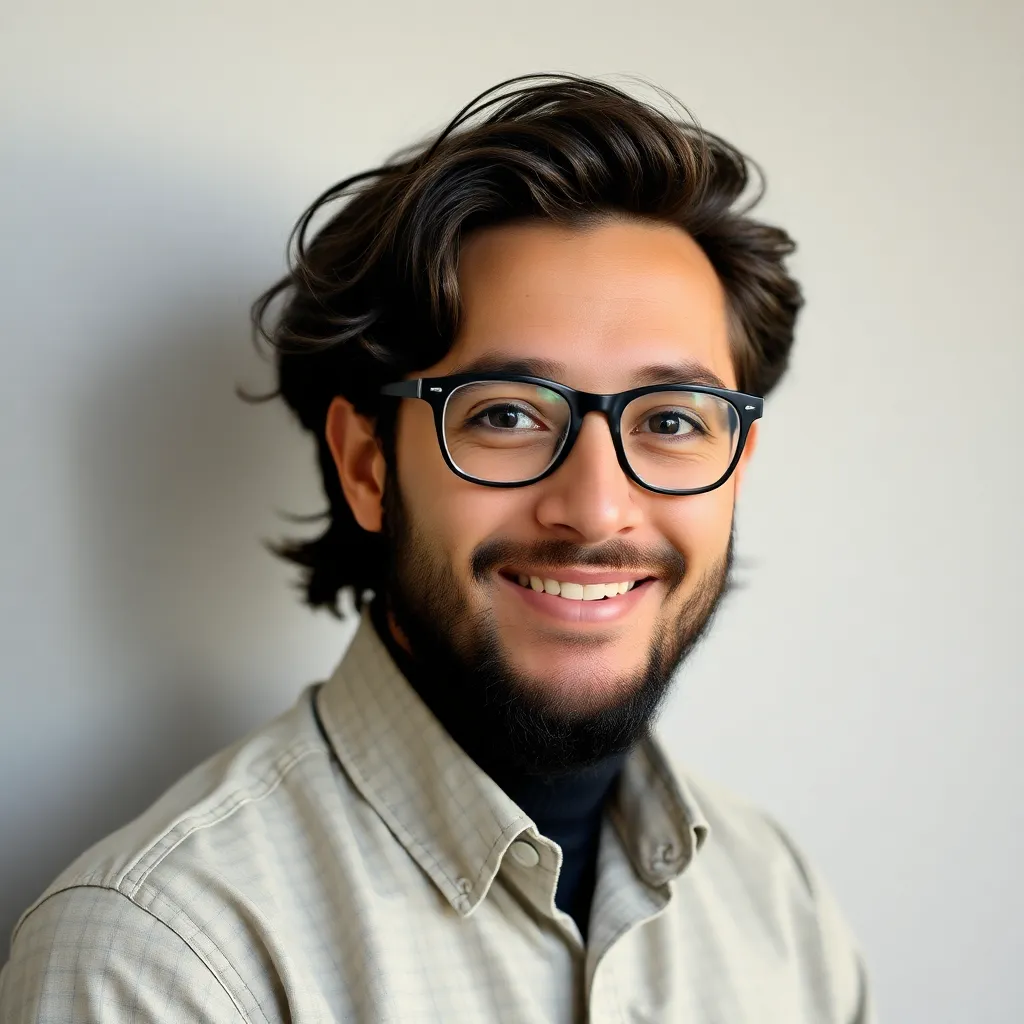
Treneri
May 13, 2025 · 6 min read
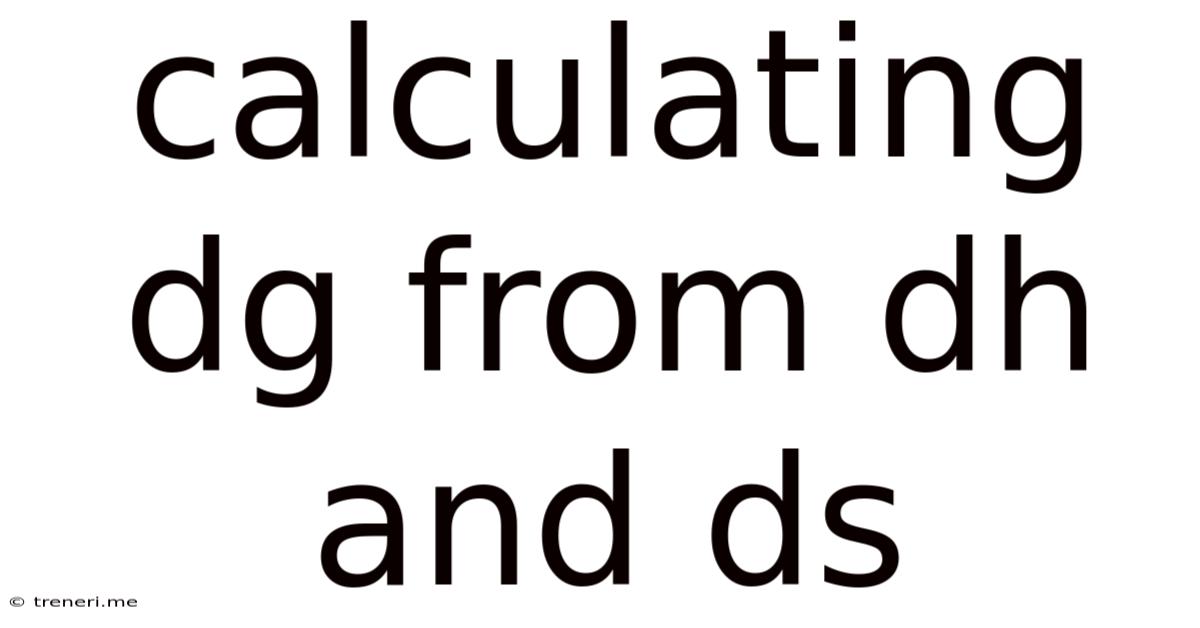
Table of Contents
Calculating ΔG from ΔH and ΔS: A Comprehensive Guide
Understanding Gibbs Free Energy (ΔG) is crucial in thermodynamics and chemistry, as it predicts the spontaneity of a reaction or process. While directly measuring ΔG can be challenging, it can be readily calculated using the enthalpy change (ΔH) and entropy change (ΔS) of the system. This article provides a comprehensive guide to understanding and calculating ΔG from ΔH and ΔS, covering various scenarios and practical applications.
The Gibbs Free Energy Equation: The Foundation of Spontaneity
The fundamental relationship between Gibbs Free Energy, enthalpy, and entropy is expressed through the following equation:
ΔG = ΔH - TΔS
Where:
- ΔG represents the change in Gibbs Free Energy (in Joules or Kilojoules). A negative ΔG indicates a spontaneous process under the given conditions, while a positive ΔG indicates a non-spontaneous process. A ΔG of zero suggests the system is at equilibrium.
- ΔH represents the change in enthalpy (in Joules or Kilojoules). This term reflects the heat absorbed or released during the process at constant pressure. A negative ΔH indicates an exothermic process (heat released), while a positive ΔH indicates an endothermic process (heat absorbed).
- T represents the absolute temperature (in Kelvin). Temperature plays a critical role in determining spontaneity, as it influences the contribution of entropy.
- ΔS represents the change in entropy (in Joules per Kelvin or Kilojoules per Kelvin). Entropy measures the disorder or randomness of a system. A positive ΔS indicates an increase in disorder, while a negative ΔS indicates a decrease in disorder.
Understanding the Contributions of Enthalpy and Entropy
The equation reveals a crucial interplay between enthalpy and entropy in determining spontaneity:
-
Enthalpy-driven processes: Processes with a large negative ΔH (exothermic) tend to be spontaneous because they release heat, making the system more stable. However, a positive ΔH doesn't automatically mean non-spontaneity; the entropy term can compensate.
-
Entropy-driven processes: Processes with a large positive ΔS (increased disorder) tend to be spontaneous because they increase the randomness of the system, which is favored statistically. Even if ΔH is positive (endothermic), a sufficiently large and positive ΔS can still make ΔG negative, resulting in spontaneity.
-
The Temperature Factor: The temperature (T) acts as a weighting factor for the entropy term. At higher temperatures, the contribution of TΔS becomes more significant, meaning entropy plays a more dominant role in determining spontaneity. At lower temperatures, enthalpy's influence becomes more prominent.
Calculating ΔG: Step-by-Step Examples
Let's illustrate the calculation of ΔG with some examples, covering different scenarios:
Example 1: A Simple Exothermic Reaction
Consider a reaction with ΔH = -50 kJ/mol and ΔS = +100 J/mol·K at a temperature of 298 K (25°C).
-
Convert units: Ensure consistent units. Convert ΔS to kJ/mol·K: ΔS = 0.1 kJ/mol·K.
-
Apply the equation: ΔG = ΔH - TΔS = -50 kJ/mol - (298 K)(0.1 kJ/mol·K) = -50 kJ/mol - 29.8 kJ/mol = -79.8 kJ/mol.
-
Interpret the result: Since ΔG is negative, the reaction is spontaneous at 298 K.
Example 2: An Endothermic Reaction with Positive Entropy Change
Consider a reaction with ΔH = +20 kJ/mol and ΔS = +150 J/mol·K at 298 K.
-
Convert units: ΔS = 0.15 kJ/mol·K
-
Apply the equation: ΔG = ΔH - TΔS = +20 kJ/mol - (298 K)(0.15 kJ/mol·K) = +20 kJ/mol - 44.7 kJ/mol = -24.7 kJ/mol
-
Interpret the result: Even though the reaction is endothermic (positive ΔH), the large positive entropy change makes the reaction spontaneous at 298 K.
Example 3: The Importance of Temperature
Consider a reaction with ΔH = +10 kJ/mol and ΔS = +50 J/mol·K. Let's analyze spontaneity at different temperatures:
-
At 298 K: ΔG = +10 kJ/mol - (298 K)(0.05 kJ/mol·K) = +10 kJ/mol - 14.9 kJ/mol = -4.9 kJ/mol (spontaneous)
-
At 200 K: ΔG = +10 kJ/mol - (200 K)(0.05 kJ/mol·K) = +10 kJ/mol - 10 kJ/mol = 0 kJ/mol (at equilibrium)
-
At 100 K: ΔG = +10 kJ/mol - (100 K)(0.05 kJ/mol·K) = +10 kJ/mol - 5 kJ/mol = +5 kJ/mol (non-spontaneous)
This example clearly shows how temperature affects spontaneity. At low temperatures, the enthalpy term dominates, while at higher temperatures, the entropy term becomes more influential.
Beyond the Basic Calculation: Advanced Considerations
The basic equation provides a foundational understanding, but several factors warrant further consideration:
Standard Gibbs Free Energy Change (ΔG°)
The calculations above use actual values of ΔH and ΔS. However, we often use standard Gibbs Free Energy changes (ΔG°), which are calculated at standard conditions (usually 298 K and 1 atm pressure). Standard values of ΔH° and ΔS° are often tabulated, facilitating calculations.
Temperature Dependence of ΔG
The equation assumes ΔH and ΔS are constant with temperature. This is an approximation. In reality, both ΔH and ΔS can vary with temperature. More accurate calculations require integrating over a temperature range, using heat capacity data. This introduces more complexity and typically requires numerical methods.
Non-Standard Conditions
The equation, as presented, applies only to standard conditions. For non-standard conditions (different temperatures, pressures, or concentrations), the equation needs modification:
ΔG = ΔG° + RTlnQ
Where:
- R is the ideal gas constant
- Q is the reaction quotient
Calculating ΔH and ΔS: Experimental Methods
The accuracy of ΔG calculations depends on the accuracy of the ΔH and ΔS values used. These values can be determined experimentally through various methods, such as calorimetry (for ΔH) and statistical mechanics (for ΔS).
Applications of ΔG Calculations
The ability to calculate ΔG has wide-ranging applications across various fields:
-
Predicting reaction spontaneity: This is the most direct application, vital in chemical kinetics and equilibrium studies.
-
Determining equilibrium constants: The relationship between ΔG° and the equilibrium constant (K) provides a way to determine K from thermodynamic data: ΔG° = -RTlnK
-
Designing chemical processes: By predicting spontaneity under various conditions, ΔG calculations help optimize chemical reactions for industrial processes.
-
Understanding biological systems: ΔG is essential in biochemistry for studying metabolic pathways and energy transformations within living organisms.
-
Material science: ΔG calculations help in predicting the stability of materials and in designing new materials with desired properties.
Conclusion: Mastering the Power of ΔG Calculation
Calculating ΔG from ΔH and ΔS is a fundamental skill in thermodynamics and chemistry. Understanding the interplay between enthalpy and entropy and their temperature dependence is crucial for accurately predicting the spontaneity of a process. While the basic equation provides a valuable starting point, being aware of advanced considerations, such as non-standard conditions and the temperature dependence of thermodynamic quantities, allows for more refined and accurate calculations. Mastering these concepts empowers scientists and engineers to design efficient processes, understand complex systems, and ultimately contribute to advancements in various fields.
Latest Posts
Latest Posts
-
How To Find Radian Measure Of Central Angle
May 13, 2025
-
How Many Years Ago Was 1982 To 2023
May 13, 2025
-
How Do You Find The Perimeter Of A Cylinder
May 13, 2025
-
Bean Bag Filling For 7ft Bean Bag
May 13, 2025
-
What Is A 60 Out Of 75
May 13, 2025
Related Post
Thank you for visiting our website which covers about Calculating Dg From Dh And Ds . We hope the information provided has been useful to you. Feel free to contact us if you have any questions or need further assistance. See you next time and don't miss to bookmark.